This article seeks to present the technological development of PON (Passive Optical Network) technologies based on recommendations from the ITU-T (International Telecommunications Union – Telecommunications), starting with their origin and highlighting their physical characteristics, distances, transmission rates, wavelengths and compatibility, up until the moment at which they reached a level of technological maturity that garnered interest in their use in internal networks and structured cabling infrastructure, as part of a means of implementation that became known as POL (Passive Optical LANs). In this context, the characteristics, advantages, and benefits of these networks are presented, and their historical development through their recognition under standards for structured cabling, such as ANSI (American National Standards Institute /Telecommunications Industry Association), and manuals, such as BICSI’s (Building Industry Consulting Service International) TDMM (Telecommunications Distribution Methods Manual). Finally, important points that must be considered when implementing these networks are discussed and certain questions that sometimes challenge ICT professionals are answered, particularly those focusing on infrastructure and that are not yet accustomed to the PON mode of operation in LAN (Local Area Networks) networks. Certain pertinent issues that usually arise when comparing POLs with a traditional network in terms of infrastructure, compatibility and performance are therefore addressed.
The technological development seen over the last forty years is astonishing. New technologies have emerged, and others were consolidated, some of which were developed during the 70s and 80s. The 90s was a decisive historical moment in the field of networks, whether telecommunications or LANs. The start of the decade was marked by the launch of the first structured cabling standard, ANSI/TIA-568, which was created to standardize and organize networks’ physical infrastructure at a time in which Ethernet protocol began to receive more attention. ANSI/TIA-568’s growth was fostered by an increasingly large range of manufacturers due to the fact that it is an open standard, one that is easy to implement. Soon, all computers began to be commercialized with one or more network interfaces operating in this pattern.
While on one hand Ethernet was growing and quickly being consolidated, technologies with great potential, such as ATM (Asynchronous Transfer Mode), began to lose its footing on the market. In the early 1990s, ATM was used in transmissions with speeds that exceeded those of Ethernet, and were pretty assertive in the management and forwarding network traffic, offering striking and innovative characteristics, such as: reduced transfer delay, greater transmission reliability, and increased efficiency in bandwidth use since channels were occupied on demand according to the traffic in each connection. Furthermore, ATM made use of TDMA (Time Division Multiple Access), in which there is no fixed allocation of time intervals to sub channels. The diffusion of ATM first occurred in SDH (Synchronous Digital Hierarchy) networks at telecommunications operators, given that its use in local networks ended was not as widespread as expected. Another factor contributing to ATM’s losing ground in LAN networks was due to the performance of Ethernet, which, in addition to the advantages already mentioned, has rapidly evolved in terms of market adherence, speed and offers a much more attractive implementation cost.
With the expansion in the use of optical fibers during the 1990s, the telecommunications industry began to look for alternatives for customer service in the last mile, with a focus on reaching subscribers’ homes through the use of optical fibers. In order to provide these services, one of the alternatives considered was the use of Ethernet. Due to its characteristics, to perform these services, a Point-to-Point infrastructure would be necessary in which two single-mode fibers are used by default: one for transmission, and another for receiving signals. This would initially make it impossible to use Ethernet in this scenario due to operating costs (OPEX – Operational Expenditure), whether due to the distance (which must not exceed 10 km, in terms of application), or to maintain a remote shelter that would support a significant amount of equipment in terms of energy and cooling, since for each customer, in addition to a pair of fibers, a dedicated switch port would need to be installed at that location.
Also, during the 90s, an idea that emerged in the 80s and until then had been cast aside for various reasons, once again rose to prominence: the PON technology. This technology soon took hold and was shaped by the maturation of optical communications, increases in speed and the cost of technologies involved, which, at that time, were beginning to drop. In terms of physical implementation, the use of PON networks required simplification of network infrastructure and the use of remote telecommunications shelters, including the entire apparatus, since they would be replaced by small passive elements known as opticalsplitters, which could easily be installed in splice or termination boxes. In addition to covering a greater distance through the use of a passive network, a pair of fibers would no longer be needed given that, in this new approach, the transmitting and receiving of signals would be carried out using a single-mode fiber, through the use ofWavelength Division Multiplexing (WDM).
Networks that are implemented using PON technology essentially consist of active equipment, usually installed at the ends of the network, and passive elements, comprised of optical cables, splitters, ODFs, patch cords, among other equipment, used to implement the connection infrastructure. In the active area of the network, the equipment that interfaces with the services, which are usually connected to the aggregation layer or network core, is known as the OLT (Optical Line Terminal), and the equipment that interfaces with the user is called ONT (Optical Network Terminal/Termination). The entirety of the passive part of the network, which is positioned between OLT and ONTs, is referred to as the ODN (Optical Distribution Network) and is a Point-to-Multipoint fiber infrastructure, built through a tree topology, due to the presence of optical splitters. For example, in an FTTH (Fiber-to-the-Home) network, the OLT is the head end installed on the operator side, while ONTs are installed inside the homes receiving service. The ODN includes the entire optical infrastructure that provides connection between the head end and the ONTs installed in these homes. Traffic generated in the layer above the OLT and sent towards the ONTs is called downstream. On the other hand, user-generated traffic existing below the ONTs, and sent towards the OLT is referred to as upstream. The figure 1 presents the described scenario for the PON technology:
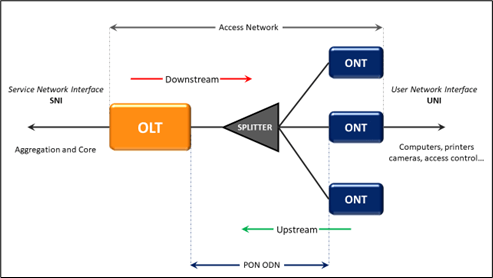
Figure 1. PON Network scenario.
In order to attend to PON networks’ specific transmission characteristics, the first development that was implemented was based on ATM and originally known as A-PON. As the name itself suggests, its implementation introduced various positive characteristics under this protocol. Installations were carried out using ATM PON and referred to as B-PON (Broadband PON), which was later standardized and released by ITU-T as a series of G.983 recommendations in 1998. Initially this standard defined transmission at speeds (bit rates) of 155 Mb/s for upstream and downstream. However, rates of 1244 Mb/s for downstream and 622 Mb/s for upstream were later adopted.
Throughout the years that followed its launch, this standard was further developed and given the capacity to transport telephony services such as POTS (Plain Old Telephone Service) and VoIP (Voice over Internet Protocol). Upstream transmission capacity was also improved through the use of DBA (Dynamic Band Allocation), which was defined and standardized.
In 2003, ITU-T released the series of recommendations G.984 known as GPON (Gigabit-PON), which defines and implements a new protocol developed based on the strengths and characteristics of B-PON, initially establishing the ATM as an optional protocol. From among the various advantages offered by this new protocol, the possibility of coexistence with upcoming generations of PON technologies and a consolidated level of convergence can be highlighted. In other words, GPON was designed to deliver/transport any telecommunications services that are necessary. GPON has been standardized to work with downstrem speed of 2488 Mb/s and 1244 Mb/s for upstream, a capacity that is shared between ONTs. GPON meets the needs and characteristics of a gigabit network and can initially accommodate up to 64 ONTs (split ratio 1:64) per OLT port at a distance of up to 20 km. The maximum distance supported is 60 km, and a maximum of 128 ONTs can be installed (split ratio 1:128), which will depend on the power budget of the optical network and the capacity, in terms of power, of the OLT transceiver (transceiver), which is determined by classes. For example, a Class B+ transceiver has a maximum range of 20 Km and the capacity to manage up to 64 ONTs, while a Class C+ transceiver has a maximum range of 60 Km and the capacity to manage up to 128 ONTs. Furthermore, with regards to applicable distances, it is important that two related characteristics be highlighted: the maximum fiber distance, and the maximum differential fiber distance. The maximum fiber distance is the maximum range between the OLT and an ONT. The maximum differential fiber distance is the difference between the range of two individual ONTs relative to a single OLT PON port. For GPONs, this distance provides a conventional range of 20Km due to PMD (Physical-Media Dependent) and TC (Transmission Convergence Layer) requirements. As a result, if a single ONT is connected to a maximum fiber distance of 60 km, all remaining ONTs installed at the same PON interface must be connected within a distance of 20 km. In this scenario, in order to guarantee proper operation of all ONTs, the minimum fiber distance used must be 40 Km in relation to the OLT. Figure 2 shows the relationship between the maximum fiber distance and the maximum differential fiber distance in this example scenario:
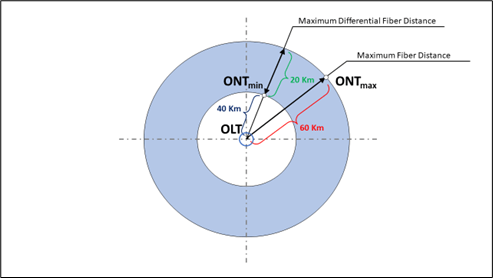
Figure 2. Example of the relationship between the maximum fiber distance and the maximum differential fiber distance.
A large number of GPON networks were installed in the years following its launch, particularly between 2008 and 2009, and continued to expand in subsequent years. Since GPON is designed to coexist only with upcoming generations of PON networks, coexistence with B-PON networks is not possible due to the use of the same wavelengths for transmitting and receiving, which operate within a window of 1490nm for downstream traffic and 1310nm for upstream traffic, respectively.
Developments picked up pace in terms of the use of PON networks over the course of the following decade, with the release of the G.987 series of recommendations in 2010, known as XG-PON (10-Gigabit-capable passive optical networks), in which X refers to the Roman numeral 10. This network was previously referred to as XG-PON1, but this term was discontinued and replaced by NG-PON1. This standard offers asymmetric transmission and reception rates totaling 9953 Mb/s for downstream traffic and 2488 Mb/s for upstream traffic. These networks operate within a distinct range of GPON wavelengths, which 1575nm ~1580nm and 1260nm~1280nm are used for downstreamand upstreamapplications, respectively. Like the parameters defined for GPON, the maximum fiber distance of 60km is supported by NG-PON1. However, the maximum differential distance defined under its scope is now 40km, something that was also defined for GPON, albeit years after its launch, through means of recommendation G.984.7. The split ratio supported by XG-PON is 1:256, which means that the protocol has a capacity of up to 256 ONTs per OLT PON interface.
In 2013, the ITU-T G.989 series of recommendations, known as NG-PON2 (40-Gigabit-capable Passive Optical Networks) was released. These documents offered characteristics used to develop the generation 2 of PON technology, based on TWDM (Time and Wavelength Division Multiplexing), as well as an optional WDM – PtPWavelength Division Multiplexing (PtP WDM) point-to-point connection system. TWDM is a PON solution that uses a multi-wavelength channel architecture, in which each wavelength is shared between multiple ONTs. PtP WDM is a PON solution that provides a specific wavelength for each ONT in both downstream and upstreamapplications, which may overlap with those of TWDM, and offers bidirectional data transmission capability between OLTs and ONTs. NG-PON2 offers a nominal aggregate capacity of 40 Gbit/s for downstreamapplications and 10 Gbit/s for use with upstream. Wavelengths were based on a plane of wavelengths for TWDM and PtP WDM in order to allow for coexistence with previous patterns as part of any migration that may eventually occur. In TWDM, the 1596nm ~ 1603nm band was reserved for downstream transmissions, and a series of options for upstream bands were made available, which were divided into Wideband, 1524nm ~ 1544nm, Reduced Band, 1528nm ~1540nm, and Narrow band, 1532nm~1540nm. Bands of 1524nm~1625nm were defined for PtP WDM, which is known asexpanded spectrum. This spectrum may be used as long as it does not interfere with TWDM or legacy systems and insulation requirements are fulfilled. Another range can be used whenever PtP WDM is used in conjunction with TWDM. This range is known asShared spectrum and uses wavelengths of 1603nm ~1625nm. The maximum fiber distance and the maximum fiber differential distance supported are the same as those defined for NG-PON1. However, the minimum split ratio must be 1:256 in such networks.
In 2016, ITU-T recommendation G.9807.1 was released, which established definitions for XGS-PON (10-Gigabit-capable Symmetric Passive Optical Network), a technology that makes use of symmetric rates, with speeds of 9953 Mb/s for both downstream and upstreamand is able to use the same wavelengths as an existing XG-PON system, or use GPON wavelengths, thus supporting both migration and coexistence with these technologies, as seen with NG-PON2. An important highlight with regards to XGS-PON’s characteristics was the maximum reuse of previous ITU-T PON recommendations. In other words, this standard’s physical transmission specifications (PMD) were derived from XG-PON, and its logic is based on NG-PON2 and XG-PON’s TC layer. Interestingly, all transmission rates for PON technologies are based on standard SDH rates.
A very important development in the evolution of PON technologies was the special interest they received for indoor plant applications, which was mainly due to their maturity, robustness, and operating characteristics. The concept of POL networks, which was the named given to the implementation of PON technology in local networks, emerged in North America around 2010. APOLAN (Association of Passive Optical LAN) was created in 2012 and is an organization that brings together manufacturers, distributors, integrators, and consulting companies, with a focus on market education and encouraging the adoption of passive optical technology in local networks. In 2014, the 13th edition of the TDMM manual was published by BICSI, which offered recommendations for the use of PON networks within buildings, in which the standards and concepts of structured cabling are applied. Up until that moment, this emerging trend was being discussed by the various committees established at regulatory entities and was officially recognized in the fourth revision of generic standard ANSI/TIA-568.0-D for structured cabling, as well as in the fourth revision of the standard for structured cabling in commercial buildings, ANSI/TIA-568.1-D, both of which were released in 2015. The following aspects can be highlighted under this revision: alignment with international standards for structured cabling, ISO 11801, and the recognition of PON technologies in Annex C of ANSI/TIA-568.0-D, including the maximum distances supported and the minimum and maximum attenuations of single-mode fiber optic channels used for PON applications. Additional characteristics related to the centralized optical cabling used by these applications were also highlighted. At the time at which this article was written, the fifth revision (revision E) of current ANSI/TIA standards have been released, and the 14th edition of the TDMM manual is available.
Cabling standards are designed for the purpose of physical hierarchal implementation of the network in star topology due to the fact that these networks are widely-accepted, flexible and easily administered. With the use of PON technology in local networks, its original tree topology can be perfectly adaptable to the topology proposed under these standards through the use of several structured cabling components, which guarantees compatibility with current implementations and future expansions, making PON networks a disruptive technology ideal for use in this type of environment. From among the main advantages of using PON technology in local networks, we can highlight a reduction in equipment costs and, in terms of the cost of the electrical energy used by equipment and cooling systems, reductions in the space occupied by cabling and equipment, total plastic consumption, total time required for installation and implementation of the technology, and the promotion of a future-proof infrastructure.
The term ‘future-proof’ is used because it characterizes the optical infrastructure used in structured cabling, which must be certified and may have a 25-year warranty provided by the manufacturer. There is therefore an interest on the part of network clients in implementing a technological update. For example, when replacing GPON with XGS-PON, only the active network equipment (OLT and ONTs) need to be replaced, and technicians do not need to be concerned with touching the installed optical infrastructure. With these characteristics, in addition to being a sustainable solution, POLs contribute to a reduction in total implementation costs (CAPEX), and in particular a significant reduction in operating costs (OPEX), which has a positive impact the total cost of ownership (TCO) throughout the network lifecycle.
Distances in LAN networks, or even Campus Area Network(CANs), are smaller if compared to those seen in ISP or Telecom Operator’s network., and therefore are not a cause for concern in terms of POL project designs. On the other hand, in order to add value to the efficiency that PON technology provides, and to address the complexity of the various services involved in a LAN/CAN network, the equipment chosen must be sufficient in offering increased capacity in terms of memory management, processing and frame forwarding. Additionally, they must be transparent to current and future applications and offer features that can be adapted according to the complexity of the project, whether in terms of redundancy (in PON interfaces, uplink Ethernet interfaces, or in terms of energy), security, centralized management, or synergy and adaptability within the existing network architecture. For example, the connection between OLT and Core can be established through means of IPv4 or IPv6 routing.
Despite the wide range of advantages provided by a POL network, there are still some concerns regarding operation of this network when compared to a traditional network in terms of infrastructure, compatibility and performance.
How can cabling performance be guaranteed within the context of infrastructure? Adequate infrastructure is guaranteed through the use of quality materials that are properly sourced, and skilled labor. Whenever all components of the optical structured cabling infrastructure originate from the same manufacturer and are installed by an Integrator company that is accredited by that manufacturer, as well as in adherence to high-level, standards-based, and certified quality control parameters, the manufacturer will be able to provide a 25-year warranty certificate. And what would the compatibility of services that are usually based on Ethernet look like in a POL network? In this context, PON technologies are exclusively implemented between the OLT and ONTs in the access network. As presented in the figure 1, the connection between the OLT to the service network — SNI (Service Network Interface) — and devices to the ONT interfaces, which is known as UNI (User Network Interface) is essentially carried out over Ethernet. As a result, various devices, such as computers, phones, printers, cameras, access controls, among others, may be connected and operated normally in a POL, in the same way they are used in a traditional Ethernet-based network. The access network (PON) operates transparently during communication between these devices and is active in transport of the Ethernet protocol between ONTs and the OLT. This transport occurs through the encapsulation of Ethernet frames in GEM (G-PONEncapsulation Method) or XGEM (XG(S)-PON Encapsulation Method), which are only visible between the OLT and ONTs.
And what would the performance and transmission speeds look like in this case? In a traditional network, devices are individually connected to switches located in the technical rooms on each floor in a commercial building through means of structured cabling, for example. Given the characteristic network use seen in these devices, the available bandwidth generally remains idle since traffic cannot be completely determined and varies according to the time and application profile used by the user. This means that bandwidth consumption may be characterized by usage peaks that occur at random times rather than in a steady fashion. It is precisely this need to address idleness in the network that POL technologies have been increasingly used and are expanding across the different markets in which they are implemented. This intelligence is based on an intrinsic QoS (Quality of Service) feature used by the OLT to manage traffic on a PON network. OLT makes use of theDynamic Bandwidth Assignment (DBA), with which it assigns real-time bandwidth between multiple ONTs connected along a single PON port according to the load offered by each class of traffic seen in each ONT. The upstream traffic for each ONT is transmitted in the form of bursts at predetermined time intervals (TDMA), which must be authorized by the OLT. This authorization is orchestrated by the OLT through means of bandwidth maps (BWmap – Bandwidth Map), which are broadcast to ONTs and used to control the time and total size of bursts. The timing and addressing of the bandwidth map determines which ONT will transmit a burst, when it is transmitted, and for how long the burst will last. Available bandwidth is therefore managed by the OLT in an efficient and intelligent manner, maintaining the expected performance for a gigabitor10 Gigabit network. In addition to these characteristics, another point that should be noted is the number of ONTs that are connected to a single PON interface. The profile of user traffic and applications must be studied in each case in order to maintain a balance between the cost of implementation and future bottleneck problems that may affect network performance due to errors committed during the design phase. The aspect that must be observed is therefore the split ratio (splitter), which physically determines the maximum amount of ONTs per OLT interface. In general terms, a 1:32 or 2:32 split ratio (whenever there is redundancy in PON ports) may be used for a GPON application in the context of passive optical structured cabling installed in commercial buildings. In critical applications that require excessive bandwidth consumption over an extended period, a smaller split ratio must be adopted. However, it is always recommended that a proof of concept (PoC) be prepared. Another point is to be aware of the minimum attenuations determined by the standards for each PON technology, because if the split ratio is too low, it may imply the use of attenuators in the OLT’s PON interfaces.
Furukawa Solutions offers a complete solution for passive indoor optical networks (POL) known as Laserway. This solution consists of elements of optical structured cabling, in addition to active equipment (OLT and ONTs) and software for simplified provisioning and management of the entire PON network, as well as support, which may include pre-sales up through specialized consulting of Application Engineering and high level design drawings prepared by the Design Engineering. After-sales support is also provided through technical Support and Implementation Engineering, which may participate in the network start-up, as well as with potential incidents occurring during the contracted period, with remote support available 24 hours a day, 7 days a week, in Spanish, English and Portuguese.
Using the Laserway solution, it is possible to implement scalable optical infrastructure made up of structured cabling, which can be certified with a 25-year warranty and is prepared for future expansions and the use of new PON technologies. Expansions may occur in an organized manner, since the infrastructure is implemented based on structured cabling standards, which offer scalability. In the case of new PON technologies, the same cabling infrastructure may be used by simply replacing the active equipment (OLTs and ONTS) located at the extreme ends of the network. Since support is provided for coexistence between GPON and new PON technologies, it is possible to carry out technological update on demand through means of a migration process. The active equipment (OLTs and ONTs) is part of the Laserway solution, support and full warranties for the network are provided by a single manufacturer, Furukawa Electric, which offers clients greater peace of mind in the implementation of a POL network, in addition to the security that results from being able to rely on professionals with consolidated expertise and that are able to offer support throughout all project phases.
Due to the benefits offer by PON technologies and the standardization implemented under norms and standards, its potential for future use is considerable given the level of market adherence and expansion that has been occurring over the last few years. An understanding of the historical development of a technology is key in understand the manner in which implementations, improvements and updates occurred in terms of the technology’s defining characteristics. In the specific case of PON technologies that are based on ITU-T recommendations, an awareness of the fact that these technologies were consolidated mainly due to their providing for the reuse of well-established standards, added to the evolution of their most striking characteristics and advantages that can be highlighted in efficiency and performance, means an increased sense of security in an implementation process offering quality and resilience. When applied to structured cabling infrastructure in local area networks, PON technologies contribute to the establishment of high-performance connections and sustainable infrastructure. Here is to the continued success and a promising future for PON technologies and POL networks.
Samuel Graeff is the Europe Senior Applications Analyst for Furukawa Electric Group. His role includes driving Furukawa’s international growth on PON LAN and Data Center networks for the EMEA region, whilst helping build Furukawa Electric’s reputation as a thought leader in the international cabling market. Graeff has an extensive performance in GPON broadband and PON LAN applications, operating in LATAM, EMEA and SEA markets, in trainings, technical support, and business development since he started working for the group.
Bibliographic references
HOOD, Dave, TROJER, Elmar. Gigabit-capable passive optical networks. New Jersey: Wiley, 2012.
[ITU-T G.983.1] Recommendation ITU-T G.983.1 (1998), Broadband optical access systems based on Passive Optical Networks (PON).
[ITU-T G.984.2] Recommendation ITU-T G.984.2 (2003), Gigabit-capable Passive Optical Networks (GPON): Physical Media Dependent (PMD) layer specification.
[ITU-T G.984.5] Recommendation ITU-T G.984.5 (2007), Gigabit-capable Passive Optical Networks (GPON): Enhancement band.
[ITU-T G.984.6] Recommendation ITU-T G.984.6 (2008), Gigabit-capable passive optical networks (GPON): Reach extension.
[ITU-T G.984.7] Recommendation ITU-T G.984.7 (2010), Gigabit-capable passive optical networks (GPON): Long reach.
[ITU-T G.987.1] Recommendation ITU-T G.987.1 (2016), 10-Gigabit-capable passive optical networks (XG-PON): General requirements.
[ITU-T G.987.2] Recommendation ITU-T G.987.2 (2016), 10-Gigabit-capable passive optical networks (XG-PON): Physical media dependent (PMD) layer specification.
[ITU-T G.989.1] Recommendation ITU-T G.989.1 (2013), 40-Gigabit-capable passive optical networks (NG-PON2): General requirements.
[ITU-T G.989.2] Recommendation ITU-T G.989.2 (2019), 40-Gigabit-capable passive optical networks 2 (NG PON2): Physical media dependent (PMD) layer specification.
[ITU-T G.9807.1] Recommendation ITU-T G.9807.1 (2016), 10-Gigabit-capable symmetric passive optical network (XGS-PON)
ANSI/TIA-568.0-D, Generic Telecommunications Cabling for Customer Premises, 2015
ANSI/TIA-568.0-E, Generic Telecommunications Cabling for Customer Premises, 2020
ANSI/TIA-568.1-D, Commercial Building Telecommunications Infrastructure Standard, 2015
ANSI/TIA-568.1-E, Commercial Building Telecommunications Infrastructure Standard, 2020
BICSI/TDMM-13, Telecommunications Distributed Methods Manual 13th Edition.